The Hope and Fury of the Most Powerful Equation in History
One simple equation promises both catastrophic war and endless clean energy.
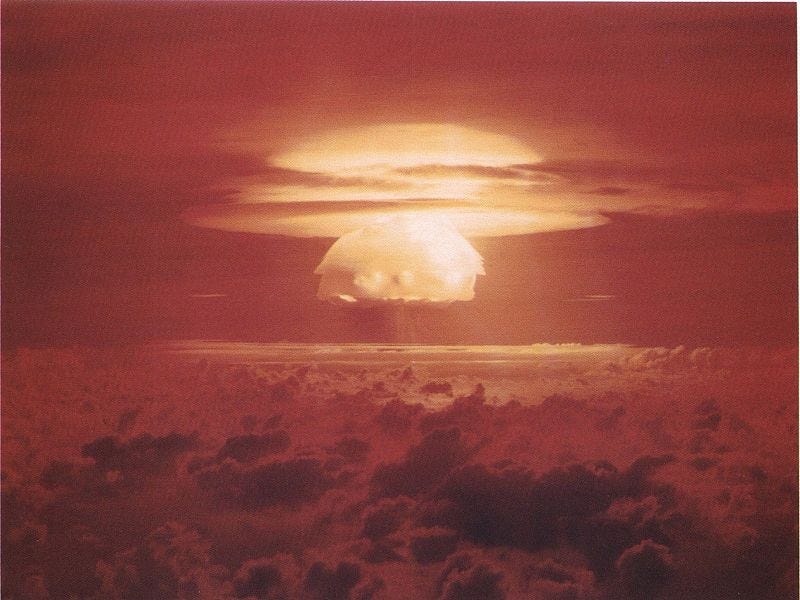
Major Andrei Durnovtsev had a problem. Slung under his aircraft — a specially modified Tupolev Tu-95 — was a bomb. That, by itself, was not much of a worry. The Tu-95, with its four powerful engines and swept back wings, was, after all, an intercontinental bomber, designed to deliver death and destruction.
But this particular bomb was no ordinary weapon. When it detonated — as it would shortly after it started falling — it would unleash the most powerful explosion ever created by Man. The shockwave, should it be used in war, was capable of destroying an entire city, or even a small country.
As it was, Durnovtsev was not heading towards a city. Instead he was flying north-east, to Novaya Zemlya, one of the most remote islands on Earth. This particular bomb would not, fortunately, be used in anger. It was a test, nominally to investigate the upper limits of nuclear weaponry, but more importantly to demonstrate the power of Soviet technology.
Even so, Durnovtsev had a problem. At 11:29 that morning, when he arrived over Novaya Zemlya, the bomb would start falling towards the island. Three minutes later it would fall through the critical altitude, four thousand metres above sea level. Then, triggered by a pressure sensor, the bomb would explode. Durnovtsev, flying at several hundred miles per hour, would be roughly thirty miles away.
That would be far enough to escape the worst of the bomb, but perhaps not enough to avoid a deadly shockwave knocking his aircraft from the sky. Andrei Sakharov, the man who designed the bomb, give him just a fifty percent chance of survival.
That, Sakharov knew, was an improvement over initial estimates. The bomb had been deliberately weakened; its power capped to lessen the blast. No pilot could hope to survive a full scale detonation; no missile would have the power to carry its full weight.
At 11:32am the bomb hit the designated altitude and exploded. The flash was spotted hundreds of miles away, in Greenland and Alaska. A mushroom cloud rose to altitudes of sixty miles, reaching the edge of space. The shockwave circled the Earth three times, spiking pressure monitors in New Zealand. Windows shattered as far away as Finland and Norway.
On Durnovtsev’s Tu-95 the heat of the blast melted and scorched layers of anti-radiation paint. Moments later the air itself shook, and Durnovtsev’s plane started to fall. By now he was seventy miles from ground zero, enough, just about, for him to ride out the shockwave. After falling thousands of feet he regained control, eventually bringing the Tu-95 down to a safe landing.
The test in Novaya Zemlya turned out to be the largest nuclear detonation in history. The bomb’s yield was somewhere around fifty-five megatons; roughly ten times greater than every bomb exploded during World War II combined. All of this energy was released in just forty nanoseconds, a flash equal to roughly one-seventieth of the Sun’s entire output.
That awesome power was made possible by a startling fact of physics. Matter, as Einstein had realised half a decade earlier, could be converted into pure energy. A single gram of matter — hydrogen, plutonium or human hair, it doesn’t matter — contains a staggeringly vast amount of energy, enough to explode with the force of the bomb that destroyed Nagasaki in 1945.
That power is encapsulated in a simple equation; one that has become perhaps the most famous equation of physics in history: E= mc². Those three simple letters capture something fundamental about our universe, something that both threatens the prospect of an Earth-shattering war, and promises a future of endless clean energy.
Physicists had long known that energy and mass were both conserved. That is, neither energy nor mass could be destroyed; only converted into other forms. Energy could be stored, for example in the chemical bonds of coal, and liberated, as we do when we burn that coal to produce heat. But energy itself could not be destroyed, or created.
This conservation of energy is a fundamental principle of physics, as is the conservation of mass. But until the dawn of the twentieth century physicists believed they were two different things — mass was always mass, energy was always energy. Einstein showed this to be wrong: mass and energy were indeed conserved, but they were also two forms of the same thing.
The implications of this were both startling and rather unsettling. If energy was really equivalent to mass, then Einstein’s equation implied that matter could be created, seemingly, from nothing. Gather enough energy into a small enough volume, then, and particles should appear as if by magic.
The reverse was more disturbing. Einstein’s equation linked energy, e, and mass, m, by a constant: c². The c represents the speed of light — roughly three hundred million meters per second. Square that number, as Einstein’s equation calls for, and you get a very big number, one with sixteen zeros.
That big number means that each gram of matter holds a tremendous amount of energy. It is thus hard to create matter. Even if we could somehow divert the entire energy output of our civilization towards producing particles, we would create just a few kilograms per year. But if we can do the reverse — convert matter into energy — we could generate incredible quantities of energy.
That was shown to devastating effect in Novaya Zemlya. Though the bomb converted only a tiny amount of matter into energy — no more than a few kilograms — it was enough to produce an explosion bigger than any other in history.
Andrei Sakharov did not watch the detonation. Instead he stayed behind at Arzamas-16, a secret laboratory buried in the Urals Mountains. Although he had been the chief architect of Durnovtsev’s bomb, he was becoming increasingly uncomfortable about the terrible power he was unleashing.
This bomb, he felt, was simply too big. No missile could carry it, which was why Durnovtsev needed to drop it from an aircraft. But he also couldn’t guarantee the pilot would survive the shockwave — the odds he placed on Durnovtsev’s survival were fifty percent, and that, perhaps, was optimistic.
Worse still was the potential fallout from the explosion. Sakharov worried that an even larger bomb might spread radioactive debris across the atmosphere, rendering large areas of the globe uninhabitable. This, however, was Soviet Russia, and those who spoke out often faced awful consequences.
Sakharov instead directed his energies to supporting nuclear treaties, and to finding peaceful applications for the technology. E=mc² allowed the possibility of terrible bombs, true, but it also hinted at the prospect of almost unlimited clean energy. Nuclear technology could lead to the destruction of mankind, but it could also be its saviour.
The result was the Tokamak, a device that Sakharov believed could harness the power of the hydrogen bomb. It was, essentially, a large chamber formed in a shape known as a torus; something rather like a doughnut. Intense magnetic fields shaped and controlled the superheated material — a plasma — formed by the nuclear reactions.
In theory the Tokamak could sustain the nuclear reaction, transforming the energy of the bomb into a clean and almost endless source of energy. In practice, however, things turned out to be harder. Plasmas are delicate things, and quickly become unstable, bringing the reaction to an end. Predicting exactly how they will behave is tricky — which makes it hard to design a working reactor.
Sakharov’s initial design did, however, partially succeeded in its goals. An experimental Tokamak reactor — codenamed T1 — was built under conditions of top secrecy. It worked, at least well enough to contain and control the plasma. But it, like every other fusion reactor ever built, failed to generate more energy than was needed to start the reaction.
Nevertheless, and despite decades of setbacks, scientists and engineers continue to pursue the holy grail of nuclear fusion. A new reactor, a Tokamak far bigger than even Sakharov could have imagined, is now under construction in France. It may, finally, produce a self sustained nuclear fusion reaction.
If it does, it would be an incredible prize, one well worth the twenty billion dollar price tag. With the almost endless power promised by Einstein’s equation the world could be transformed — the threat of energy shortages and carbon pollution made a thing of the past.
For Sakharov, however, the bomb represented a turning point. He increasingly spoke out against the Soviet leadership, and against nuclear weaponry. For his efforts to promote human rights he was awarded the Nobel Peace Prize, though his government refused to allow him to collect it.
Physicists, Sakharov later argued, could not be blind to the consequences of their work. They have, and had, a responsibility to speak up when they saw moral wrongs; to resist when their work was twisted to evil ends. The dreadful power of the hydrogen bomb, he knew, showed that only too clearly.
Thank you for this article. If it informs even one person of the qualitative difference between conventional weapons and atomic bombs, this article will have served.
A small issue:
Re: "Matter ... could be converted into pure energy."
Energy is not a thing; rather, it is a thermodynamic calculation which describes the behavior of things. What is called the "annihilation of mass" actually converts the mass into the behavior of other masses and electromagnetic radiation.
"A single gram of matter — hydrogen, plutonium or human hair, it doesn’t matter — contains a staggeringly vast amount of energy, enough to explode with the force of the bomb that destroyed Nagasaki in 1945." Wow. Great article.